Abstract
Astronauts on missions to the International Space Station, the moon, and beyond are exposed to several hazards, one of which is space radiation. Space radiation is composed of tiny particles from various sources that spread out in all directions throughout the solar system. These particles have a wide range of energies, and some of them are a serious concern for the safety of space crews. Radiation that passes through the shielding on spacecraft and into the human body can damage cells and may lead to the development of cancer or other negative health effects. To protect astronauts, the National Aeronautics and Space Administration (NASA) is trying to better understand the space radiation environment so they can minimize the effects of radiation exposure on astronauts. This article describes the kinds of space radiation and the approaches that NASA is using or studying to keep astronauts as safe as possible.
In space, astronauts are exposed to several health risks, including a low-gravity environment, stress from being in a small space for a long time, and limited access to health care. One of the greatest risks astronauts face is space radiation [1]. Space radiation may have high enough energy to strip electrons away from the nuclei of atoms, so it can damage human tissue and lead to numerous health problems, including cancer. Space radiation comes from several sources. The Sun ejects charged particles (called ions) into space during violent eruptions known as solar particle events (SPEs). These events may be hazardous to crew members if a SPE storm shelter is not available. Galactic cosmic rays (GCRs) are formed when the shock waves from galactic supernovae (events in which stars explode) send particles like protons and heavier atomic nuclei into space at very high energies, which may cause those particles to penetrate deeply into the spacecraft and human tissue. In addition to these sources of radiation, energetic protons and electrons may also become trapped in Earth’s magnetic field, in a region known as the Van Allen radiation belts. This article describes SPEs, GCRs, the Van Allen radiation belts, the potential health risks of radiation exposure, and methods that the National Aeronautics and Space Administration (NASA) uses to reduce radiation risks.
Solar Particle Events
Solar flares and coronal mass ejections (CMEs) are examples of SPEs where particles are ejected into space from the sun [2]. Space radiation is often described in terms of the number of particles with a specific energy passing through a specified unit of area. This is called differential particle flux. If a CME has a large flux, that means there are a large number of the particles (more radiation) passing through the area. Likewise, a small flux means a smaller number of particles (less radiation). Solar flares consist mostly of high fluxes of electrons but are usually short lived and do not cause significant health risks. In contrast, CMEs may last from a few hours to several days and consist of large plumes of plasma (hot ionized gas), mostly made up of protons. Compared to solar flares, the abundance of protons and the higher energies of CMEs can cause more damage to human tissue. Since CMEs can be predicted several hours to days before they reach a spacecraft, astronauts can protect themselves by setting up storm shelters. Solar conditions are monitored by spacecraft such as the Solar Dynamics Observatory, which can inform crew members of approaching CMEs.
You may have seen pictures of the sun on which darker areas, called sunspots, are visible. The Sun has been observed to have an 11-year solar cycle, where the solar activity transitions from a solar minimum, with few or no sunspots, to a solar maximum, with a larger number of sunspots [2]. CMEs occur most frequently during the relatively high activity of solar maximum, when more sunspots are seen. An example of a CME is shown in Figure 1. Have you heard of the northern or southern lights, also called auroras? These bright, colorful lights observed near the Arctic and Antarctic regions are caused by CMEs, as ions stream into Earth’s magnetic field and cause molecules in Earth’s atmosphere to emit light. Large CMEs can even damage satellites.
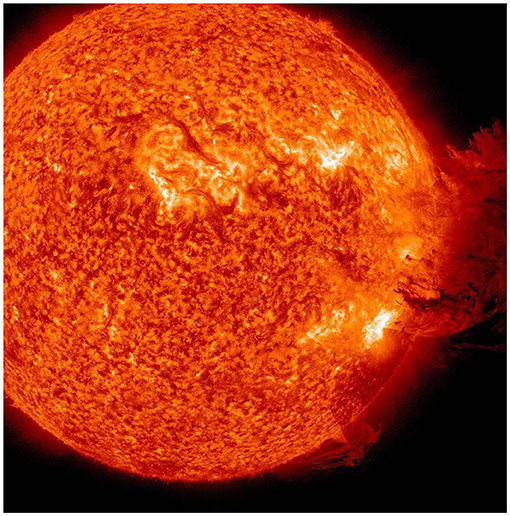
- Figure 1 - A CME captured by the Solar Dynamics Observatory on 7 June 2011.
- You can see the ejected plasma on the right side of the image, which was nearly half the diameter of the Sun! To put this into perspective, this CME was approximately 50 times the diameter of Earth (Image credit: Solar Dynamics Observatory and NASA).
Galactic Cosmic Rays
GCRs are produced from the remains of supernovae (Figure 2) [3] and consist of protons, helium nuclei, and heavier ions that can move nearly at the speed of light [2]. Supernovae are grouped into two types. Type I supernovae occur when white dwarf stars (dense stellar cores that are nearly the size of Earth) accumulate mass from a nearby star. Once the mass of a white dwarf star exceeds 1.4 times the mass of the Sun, the white dwarf explodes violently [4]. Type II supernovae occur when massive stars use all of their nuclear fuel and explode, with light intensities that may be brighter than a galaxy for a short time.
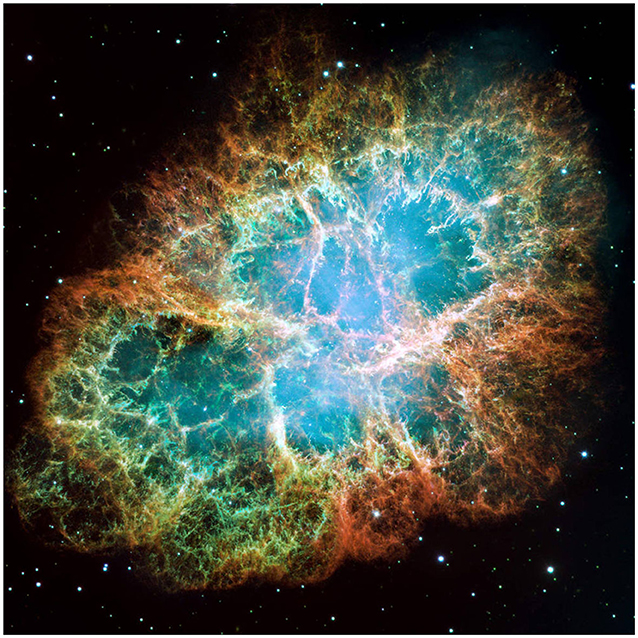
- Figure 2 - A supernova remnant known as the Crab Nebula.
- A supernova is an event in which a star explodes leaving behind mostly gases surrounded by a shockwave that accelerates protons, helium nuclei, and other particles into space at high speeds. The particles travel through space and eventually into the solar system, where astronauts may be exposed to the harmful radiation (Image credit: NASA).
GCRs vary with the solar cycle. During the solar maximum, the Sun’s magnetic field is more intense, so it deflects a lot of the GCRs, meaning that differential particle flux is decreased. During the solar minimum, the Sun’s magnetic field is less intense. There are fewer GCRs blocked, and this means there is larger differential particle flux when compared to solar maximum. The radiation risk is greater during solar minimum because astronauts receive more radiation exposure. Low energy GCR fluxes at solar minimum may be over 10 times greater than the fluxes at solar maximum. However, particles with very high energies are not as effectively blocked by the Sun’s magnetic field. These high-energy GCRs can penetrate spacecrafts and may be harmful to humans. Unlike SPEs, which happen only periodically, GCRs are always present, which makes them a major challenge for long space missions, such as a mission to Mars. During long spaceflights, astronauts could receive amounts of radiation greater than the career safety limit set by NASA.
Van Allen Radiation Belts
The Earth’s iron core creates a magnetic field around the planet that exerts a force on charged particles. In space, protons and electrons are charged particles that experience forces from the Earth’s magnetic field and become trapped as radiation belts around the Earth. The Van Allen radiation belts (Figure 3) consist of inner and outer belts: the inner belt mostly contains protons and electrons, and the outer belt contains mostly electrons [2]. The flux of electrons in the outer belt is nearly 10 times greater than the electron flux in the inner belt; however, this radiation does not penetrate deeply into spacecraft materials. The protons are more energetic and pose a greater radiation risk. The International Space Station orbits Earth at an altitude that is beneath most of the trapped proton radiation, although there is a region known as the South Atlantic Anomaly, where changes in Earth’s magnetic field increase radiation exposure from protons.
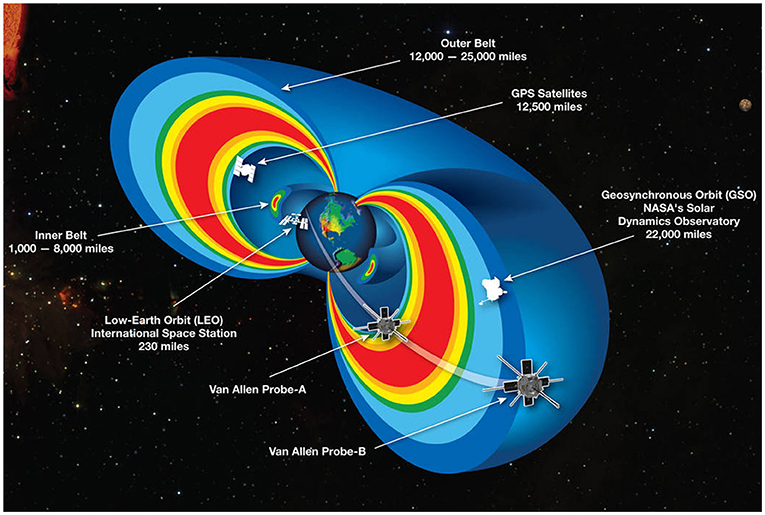
- Figure 3 - Earth’s magnetic field traps protons and electrons in layers around the Earth known as Van Allen radiation belts.
- Here, you can see the belts and the approximate locations of the International Space Station in low-Earth-orbit, probes that measure the Van Allen radiation belt, and global positioning system satellites (Image credit: NASA).
Protecting Astronauts From Radiation Risks
Space radiation may lead to many health effects such as cancer, heart disease, stroke, problems with brain function, and diseases of the brain and spinal cord. NASA uses several strategies to minimize the risk of space radiation for astronauts. These include designing spacecraft shielding to reduce radiation exposure, providing storm shelters for use during SPEs, carefully planning space missions to avoid as much radiation as possible (including timing with the solar cycle), and selecting crew members that are best suited to perform NASA’s missions. Even with these precautions, astronauts are likely to exceed their career radiation limits on long missions, such as a mission to Mars. So, NASA is also investigating whether medications can be used to reduce risk from radiation exposure. Studies have shown that some well-known medications, including aspirin and medicines to treat blood clots and diabetes, can reduce the chances of getting cancer or dying from cancer. NASA has recently shown that these medicines may also reduce the risk of cancer deaths caused by space radiation exposure [5].
Conclusion
Space contains a complex mixture of radiation, consisting of particles from SPEs, GCRs, and radiation belts, all of which can be dangerous to human health. Timing missions so that they happen during the safest part of the solar cycle is very important to keep radiation exposure to a minimum. GCRs are the hardest type of radiation to protect astronauts from; therefore, missions may be planned during solar maximum to minimize GCR exposure, even though SPEs are more likely to occur then. Crew members may decrease their radiation risks from SPEs by using storm shelters made from resources onboard the spacecraft. Astronauts onboard the International Space Station or other missions during which spacecraft stay relatively close to Earth are somewhat protected from GCRs, since Earth’s magnetic field weakens the GCR flux. NASA is working to make spaceflight safer for astronauts by taking various steps to protect them from space radiation, including potential new medicines. The aim is to protect astronauts enough that long-distance missions, such as to Mars, will eventually be possible.
Glossary
Solar Particle Events: ↑ Ejections of particles (radiation) from the sun that may be harmful to astronauts
Galactic Cosmic Rays: ↑ Protons, helium nuclei, and other particles that move uniformly through the solar system and come from supernovae located in the Milky Way galaxy.
Supernovae: ↑ Events in which stars explode and accelerate high energy particles into space.
Radiation Belts: ↑ Radiation consisting of protons and electrons that have become trapped in the Earth’s magnetic field
Solar Flares: ↑ Short-lived events of mostly electrons ejected from the sun that usually do not pose serious health risks for astronauts.
Coronal Mass Ejection: ↑ An ejection of charged particles (mostly protons) from the sun’s outermost atmospheric layer.
Differential Particle Flux: ↑ The number of particles per unit energy that pass through a specified unit of area.
Sunspots: ↑ Dark spots on the surface of the sun that are cooler than the surrounding areas on the solar surface. Large numbers of the spots are associated with high solar activity and an increased likelihood for coronal mass ejections and solar flares to occur.
Conflict of Interest
The author declares that the research was conducted in the absence of any commercial or financial relationships that could be construed as a potential conflict of interest.
Acknowledgments
This work was supported by the Human Research Program of the Human Exploration and Operations Mission Directorate of the National Aeronautics and Space Administration.
References
[1] ↑ Afshinnekoo, E., Scott, R. T., MacKay, M. J., Pariset, E., Cekanaviciute, E., Barker, R., et al. 2021. Fundamental biological features of spaceflight: advancing the field to enable deep-space exploration. Cell 183:1162–1184. doi: 10.1016/j.cell.2020.10.050
[2] ↑ Benton, E. R., and Benton, E. V. 2001. Space radiation dosimetry in low-earth orbit and beyond. Nucl. Instr. Methods Phys. Res. Section B. 184:255–294. doi: 10.1016/S0168-583X(01)00748-0
[3] ↑ Ackermann, M., Ajello, M., Allafort, A., Baldini, L., Ballet, J., Barbiellini, G., et al. 2013. Detection of the characteristic pion-decay signature in supernova remnants. Science 339:807–811. doi: 10.1126/science.1231160
[4] ↑ Woosley, S. E., and Weaver, T. A. 1986. The physics of supernova explosions. Ann. Rev. Astron. Astrophys. 24:205–253. doi: 10.1146/annurev.aa.24.090186.001225
[5] ↑ Werneth, C. M., Slaba, T. C., Blattnig, S. R., Huff, J. L., and Norman, R. B. 2020. A methodology for investigating the impact of medical countermeasures on the risk of exposure induced death. Life Sci. Space Res. 25:72–102. doi: 10.1016/j.lssr.2020.03.001